John Cioffi, CEO and Chairman of the Board, ASSIA , Inc., Peter Chow, Co-Chief Technology Officer, ASSIA, Inc., and Kenneth Kerpez, Principal System Engineer, ASSIA, Inc.
Published: 27 Nov 2017

CTN Issue: November 2017
A note from the editor:
Tired of reading articles on 5G? Well to remedy this malaise, this month we called on some of the guys who were there at the start of Digital Subscriber Line technology back in the 90s, and who are still making it happen here in the 10s, to tell us what is the latest and greatest in DSL technology and how this old man of last mile delivery is still delivering increasing data rates to the home. Comments are, as always, most welcome.
Alan Gatherer, Editor-in-Chief
DSL’s ongoing efficient enablement of the internet continues…long live DSL!
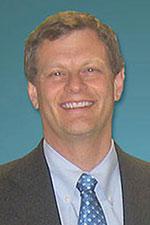
John Cioffi, CEO and Chairman of the Board, ASSIA, Inc.
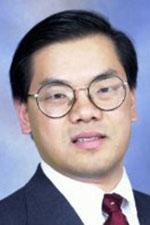
Peter Chow, Co-Chief Technology Officer, ASSIA, Inc.
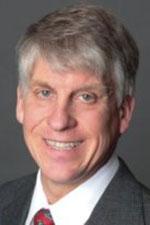
Kenneth Kerpez, Principal System Engineer, ASSIA, Inc.
Digital Subscriber Line (DSL) Internet subscriptions form an overwhelming majority (60 - 70%) of the world’s 700+ million fixed-line access connections today. While the name for any DSL link may have changed terminology to “G.fast”, “homes passed”, “fiber-to-the-curb/node/basement/distribution-point”, etc., those same copper twisted-pairs today are the workhorse delivery mechanism to billions of end-user devices and Internet endpoints in residential and commercial connections. Even though the transmission characteristics of fiber may be better, it is the cost of replacing copper by fiber that has delayed the promises of fiber to everyone’s home for over 40 years*. This article provides an update on some of the most recent developments in DSL technology.
Almost half the DSLs today no longer connect a central office all the way to a residence, but instead connect a point in the outside telephone plant to which fiber could be economically deployed to a home. Those intermediate aggregation points and/or deployment topologies are known by many names; such as, fiber-to-the-X (FTTx), where X = “node”, “basement”, “curb”, “distribution point”, etc., or “optical network unit (ONU)”. These FTTx systems are often touted as “fiber” connections in the press and by the operators or internet-service providers (ISP), but they very much are still “DSLs”. The fiber deployment programs fell short of their promise because of the high cost of construction and deployment, which runs at an average cost of about$3,000 / home connected. Whereas, the case for a short twisted-pair link in conjunction with fiber can be easily 10x less costly on average over the same connections, delivering similar internet speeds.
Figure 1 shows a plot of achievable data rate versus twisted-pair copper loop length. Clearly, as the length of copper is shortened, the achievable DSL data rates reach or exceed those offered by the best promised fiber-to-the-home systems. The cost of the fiber to an intermediate point is shared over all the subtended users, while the cost of a fiber to each home cannot be so shared. There are over 1.3 billion phone lines globally today, with roughly 500 million of them today carrying digital data / internet via DSL signals. The cost to replace them all with fiber would exceed 1.5 trillion US dollars.
Twisted-pair phone lines (invented by Alexander Graham Bell himself in 1878) were not originally intended for digital data transmission because they have highly variable signal attenuation and noise characteristics from line to line (and those characteristics also vary with time). A key development was the use of multi-carrier modulation using a self-learning artificial-intelligence method known as “loading” and supplemented by a “bit-swapping” technology first invented in [2]. The fundamental innovation was changing the transmitter modulator according to the channel learned and tracked at the receiver (which would be sent back to the transmitter through a reverse highly reliable control / overhead channel). This basic concept is still used today in all DSL technologies delivering higher and higher connection speeds.
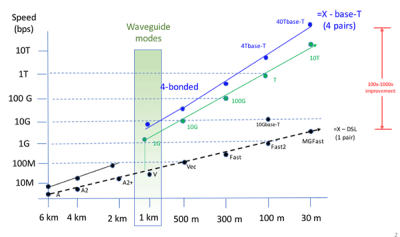
The original ADSL1 (A = asymmetric) systems, deployed at the beginning of this century allowed speeds up to 8 Mbps over several kilometers of copper twisted-pairs, were followed by ADSL2+ systems that increased speeds to (up to) 25 Mbps [3]. VDSL (V = very high-speed) then became widely deployed from 2008 – 2012 time frame at speeds up to 50 Mbps [4]. Advanced DSL systems became limited by the crosstalk from other DSLs as the speeds increased (and the loop lengths shortened), and a second major DSL artificial-intelligence innovation called “dynamic spectrum management (DSM)” or “vectoring” was introduced to remove this crosstalk [5]. Vectoring is the origin of the so-called MU-MIMO or Massive-MIMO systems perhaps better known (and later adopted) in wireless communication, which required a new level of artificial intelligence and learning over all the transmitters between an entire neighborhood of phone lines. Vectoring technologies allow speeds up to several hundred megabits/second (on each residential connection independently). This is better; i.e., delivering higher data rate to each individual end user, rather than delivering one aggregated speed that must then be shared by many customers as in the cases of fiber PON, cable, and wireless systems. In aggregate, for example, a neighborhood twisted-pair bundle with a vectored set of 100 DSLs actually carries a higher data rate than a GPON system (and certainly more than a DOCSIS 3.1 system) would to the same homes covered.
As (non-PON) fiber systems improve speeds to the fiber/copper interface, new DSL standards known as G.fast, 212 MHz G.fast, and G.mgfast increase speeds up to 800 Mbps, 2 Gbps, and 5-10 Gbps, respectively [6]. G.mgfast should encompass point-to-multipoint transmission to many in-home devices, and if there are 2 or more twisted pairs to the home the so-called phantom-vectoring methods can be used to transmit 10’s of Gbps.
There have been many announcements this year of 500 Mbps to 1 Gbps “DSL” services (often under names not using the acronym DSL) by AT&T, Deutsche Telekom, British Telecom, Swisscom, NBN Co, and many other service providers [7].
Finally, theory based on some limited available measurements have recently found that more than 1 Tbps of data speed, per twisted pair, may be possible in the future using the higher-order waveguide modes over a cable of twisted pairs, along with very powerful vectoring/MIMO processing. These methods may be used to provide 100’s of Gbps, far more economically, to broadband users and the 10’s of millions of global ``small cells’’ necessary for the next 5G generation of wireless systems to reach their full potential economically [8]. More measurements should help verify or alter these projections, but it seems that DSLs, based on good-old Alexander Graham Bell’s twisted pairs, may indeed have another 40 years or more of contribution to our evolving and ever expanding use of the Internet.
* Probably the first country to promise, and then not deliver, fiber to all homes was the United States in its “Information Superhighway” or “National Information Infrastructure (NII)” initiatives during the mid-to-late 1980’s, and it was followed in the subsequent decades by many other nations that increased the amount of fiber deployed but did NOT reach more than a small percentage (< 10%) of their homes with fiber directly [1].
References
- https://en.wikipedia.org/wiki/Information superhighway.
- “Method and Apparatus for Adaptive, Variable Bandwidth, High-Speed Transmission of a Multicarrier Signal over Digital Subscriber Lines,” U.S. Patent 5,479,447, P. Chow and J.M. Cioffi, 26 December 1995.
- ITU-T G.992.3, Asymmetric digital subscriber line transceivers 2 (ADSL2), 2009/
- ITU-T G.993.2, Very high speed digital subscriber line transceivers 2 (VDSL2), 2015
- ”Dynamic Digital Communication System Control,” U.S. Patent 7,158,563, G. Ginis, W. Yu, C. Zeng, and J. Cioffi, January 2, 2007.
- ITU-T G.9701, Fast Access to Subscriber Terminals (FAST) – Physical layer specification, 2014.
- See any of https://www.ispreview.co.uk/index.php/2017/08/openreach-extend-330mbps-g-fast-broadband-pilot-1-million-uk-premises.html, https://www.swisscom.ch/en/about/medien/press-releases/2016/10/20161018-MM-Gfast.html, http://gfastnews.com/index.php/90-r/381-2019-deutsche-telekom-g-fast,&n…;https://www.nbnco.com.au/corporate-information/media-centre/media-releases/announcement-for-gfast-launch.html
- Terabit DSLs. J.Cioffi, K. Kerpez, C. Hwang, and I. Kanellakopoulos, 2017 G.fast Summit, Paris, May 2017 and also TNO Conference, The Hague, June 2017, or full paper at web site: https://www.assia-inc.com/terabit-dsl/.
Statements and opinions given in a work published by the IEEE or the IEEE Communications Society are the expressions of the author(s). Responsibility for the content of published articles rests upon the authors(s), not IEEE nor the IEEE Communications Society.