Aryan Kaushik, University of Sussex, UK and Muhammad Zeeshan Shakir, University of the West of Scotland, UK
Published: 4 Jan 2023

CTN Issue: December 2022
A note from the editor:
For the last article of 2022 we are exploring yet another perspective on Non-Terrestrial-Networks. The last two months the authors explored some interesting applications of NTN based communications networks, this month the authors acknowledged the great potential that these networks, when paired with terrestrial networks can have, while at the same time providing a perspective regarding the type of technologies that are needed to make the deployment and overall performance of these “3D” networks as efficient and reliable as possible. Of particular interest is the subject of smart surfaces and what they can do to aid the overall performance of 3D networks. Smart surfaces (a.k.a. RIS) have been and will continue to be the subject of extensive research and debate with many challenges remaining in the quest for implementable solutions. In the end, there’s no debating that sky/space-based networks in their many forms are already here, and the industry will continue to address many open issues; perhaps 6G provides the optimal landing spot but 5G is already providing the runway needed for them to take off.
As we close 2022 and launch a new year, the CTN editorial board sends its best wishes to all its readers and look forward to an exciting 2023.
Miguel Dajer, CTN Editor-in-Chief
Non-Terrestrial Networks: Have We Found that Ultimate Catalyst for Global Connectivity in 6G?
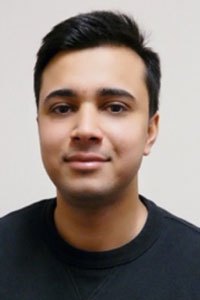
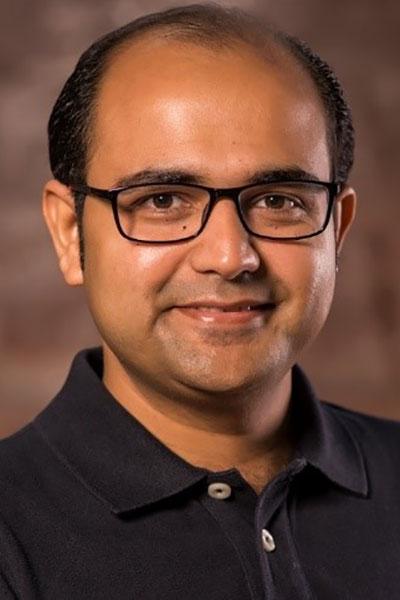
Introduction
The sixth generation (6G)-envisioned non-terrestrial networks (NTNs) will provide a key paradigm shift for wireless standardization. It is expected that mobile connectivity will constitute over 70% of the global population, and fifth generation (5G) connections are estimated to consist of over 1.4 billion mobile devices by 2023 [1]. By 2027, 5G subscriptions will be 4.4 billion representing faster growth than previous generation standards, with average monthly usage per smartphone reaching to 40 GB globally, and 52 GB of data traffic in both Western Europe and North America alone [2]. Reference [3] provides a complete overview of the ongoing global activities in 6G mobile communications, next generation technology enablers, recent trends, use cases and networks requirements towards the year 2030. The currently standardized 5G and 5G Advanced systems and networks are being offered to mobile consumers with smart-phones, however there is an expected gradual shift towards fulfilling the enhanced user demands which utilize massive internet-of-everything (IoE) and massive machine type communication (mMTC) devices. Along with this, the global push of the United Nations (UN) and its country members to close the digital divide will provide ample fuel for NTN based use cases. The enhanced demand of anything, anytime and anywhere connectivity, or fast and continuous service, can be driven by the “not so unconventional anymore” NTNs in a futuristic yet robust manner.
NTNs: Where Are We with Current Research Trends & Challenges?
A general NTN framework may consist of different driven units including traditional satellites, starnet, unmanned aerial vehicles (UAVs) and other flying platforms. The advanced enablers in NTN frameworks tend to bring the space serving units closer to the Earth which as a result reduces latency, power requirement, and the size of the units, although with restricted serving areas. For instance, SpaceX’s project Starlink is set to place around 42,000 satellites at an altitude of 200-400 miles by the end of this decade. In this direction, the optimal and efficient design of NTNs can tackle the following: a) large transmission delays and power requirements due to high altitude, b) limited serving capability owing to the restricted spectrum and large attenuation over high frequency deployments such as millimeter wave (mmWave) through to terahertz (THz) spectrum. Therefore, evolving circuit techniques, modern signal processing methods, machine learning (ML) and artificial intelligence (AI), edge and cloud computing, satellite doppler-effect exploitation, reconfigurable intelligent surfaces (RIS), wireless backhaul, automated UAVs for aerial communication, coverage hole detection using UAVs, and the use of UAVs as relays in IoE networks for disaster management etc., have drastically changed the realization of the next generation NTNs.
The overview, use cases, deployment scenarios and various architectures on 5G-envisioned NTNs are provided in [4]. In terms of the Third Generation Partnership Project (3GPP) work on the evolution of the 5G technologies, there has been a recent focus on NTNs for their latest release, i.e., Rel-17, whereas Rel-16 addressed the enabling 5G New Radio (NR) support for NTN technology including architecture, higher-layer protocols and physical layer (PHY) aspects [5]. The current trends of NTNs in 5G, 5G Advanced and 6G-envisioned communications is highlighted in [6]-[8]. Reference [6] focuses mainly on the NTN technology being envisioned for 6G systems and not on the standardization perspective, [7] provides a comprehensive survey on NTNs and briefly covers 3GPP-oriented NTN, whereas [8] addresses new research trends and applications in the field of NTNs.
The demand for evolution of NTN based communication also arises in remote areas, including forests and deserts, where it is still difficult to provide high coverage [9], [10]. The advanced technical approaches in NTNs enable possible resources and solutions towards these issues such as aiming for hardware-efficient NTNs which reduce deployment cost against conventional satellite networks. Accordingly, and given the high degree of activity in the private industry, standardization bodies and academic research we can venture to state that upcoming wireless generations will be stepping into the true wireless world leveraging the full potential of 3D type network which include terrestrial plus NTNs.
NTN technology encompasses anything non-terrestrial such as satellite communication networks which include low Earth orbit (LEO) satellites (with altitude of 500 to 1,200 kms), medium Earth orbit (MEO) satellites (with altitude of 5,000 to 20,000 kms) and geosynchronous Earth orbit (GEO) satellites (with altitude around 36,000 kms). NTNs also consist of high-altitude air platforms (HAPs) including air-borne and sky-oriented systems such as airplanes, airships, (futuristic) flying cars, and UAVs, all with the capacity of providing wireless communications. Some of the most recent studies reveal that standardized wireless frameworks can be reliably established over the LEO satellites. Although MEO and GEO satellites can provide reliable communications services, their usability in advanced communications applications is limited because of known large latency/delays and the need for higher transmission power and specialized terminal equipment which translates into higher cost. On the other hand, LEO satellites can provide service with more direct connectivity and lower latency than MEO/GEO satellites. However, LEO-oriented NTN communications still requires hardware-efficient designs and more directional beamforming-based approaches to assist the low-cost deployments and to provide more advanced solutions that meet the current and future demands of end users and service providers.
Fig. 1 (a) shows a basic illustration of satellite communication with mobile users and automated vehicles. Technical advancements in satellite communications, NTNs and future of wireless networking can be found in the 5G Infrastructure Public Private Partnership (5GPPP) vision paper [11], development of International Mobile Telecommunications (IMT) vision and overall objectives by ITU-R in [12], and recent 3GPP updates and vision on radio access network (RAN) in [13]-[15].
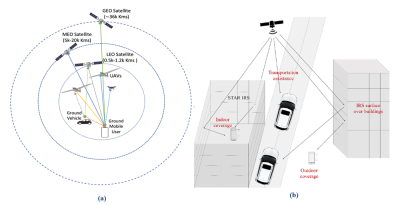
Furthermore, in terms of the latest research trends, RIS-assisted global coverage of LEO satellites present a potential paradigm shift for the next generation standards. Fig. 1 (b) presents a scenario of a LEO satellite providing massive coverage including indoor coverage (via simultaneously transmitting and reflecting (STAR)-RIS) and outdoor coverage (via RIS/IRS embedded buildings), and transportation assistance to ground vehicles, leading towards vehicle-to-everything (V2X) technology. RIS has attracted significant attention of both industrial and academic researchers in the wireless community. Recently an Industry Specification Group (ISG) on RIS has been formed by ETSI to identify use cases and deployment scenarios of RIS based systems and networks [16]. We believe that the latest technical advancements in RIS can be directly or adjacently integrated with NTNs to further enhance the overall system performance assuming that RIS type solutions can indeed be created to perform as the theory predicts. We envision that the use of NTNs in many use cases would be an industry breakthrough. Still, the on-air backbone architecture suffers from various issues including: a) limited power availability and processing ability [17], b) Large serving areas aiming to serve large number of users which restricts the deliverable rate owing to the restricted resources, c) though space-networking/NTN possesses high Line of Sight (LoS) probability as compared to the terrestrial framework, yet locations behind high-rise buildings and low altitude regions face Non-LoS (NLoS) challenges which affects the overall communication performance, d) data privacy and secrecy in space-networking/NTN added wireless communication where serving nodes are easily accessible to the eavesdroppers.
In the following section, we present a few useful considerations of the NTN technology.
Aerial NTNs
The deployment of 5G technologies including backhaul/fronthaul and access, and its standardization is a mission critical task. The latest 3G/5G Public-Private Partnership (3GPPP/5GPP) claims to be the world's biggest 5G research program and it is a joint initiative between the European Commission (EC) and the European Information and Communication Technologies (ICT) industry, which aims to deliver 5G solutions, architectures, technologies and standards. Both academia and industry have started taking keen interest in utilising flying platforms such as UAVs, drones and unmanned balloons for wireless communications. These flying platforms can be manually controlled but are mainly designed for autonomous pre-determined flights. The latest flying platforms are capable of carrying radio frequency (RF)/mmWave/free space optics (FSO) payloads along with an extended battery life [18]. On the basis of their flying altitude range, flying platforms are categorised into: i) low-altitude platform (LAP) (less than 5 kms), ii) medium-altitude platform (MAP) (between 5 kms-10 kms), and iii) HAP (greater than 10kms but less than 80 kms).
With the aim of developing an enhanced cellular support for UAV communications, 3GPP has already been gathering key industrial players, producing systematic measurements and accurately modelling of UAV-to-ground channels [19]. There is remarkable industrial and academic involvement into UAVs, LEO satellites and other flying platforms, implementing technically advanced and robust solutions such as edge computing, cloud computing, and non-orthogonal multiple access approaches for NTN [20]-[27]. Furthermore, efficient backhauling/fronthauling of either the small cell base station (SBSs) or drone-BS remains a significant challenge. One needs to consider exploring a novel airborne network with layered architecture where flying platforms are operating at various altitudes with different functionalities: drones flying at a lower altitude will offer access networks type service, and drones flying at relatively higher altitudes will offer backhaul network service. The layered airborne architecture will realise a true network densification concept, in which SBSs or mobile users can be connected to the core network through flying platforms to offer either wireless fronthaul/backhaul or access network type capabilities.
As we indicated previously, unmanned flying platforms of various types including UAVs, drones and tethered balloons are categorized into HAPs/MAPs/LAPs carrying RF/mmWave/FSO payload (transceivers) with extended battery life capabilities, and floating or moving in the air at quasi-stationary positions, with the ability to move horizontally and vertically to offer 5G and 5G Advanced cellular type access and associated support services. In terms of 6G-envisioned deployments, which will likely be a continued evolution of 5G Advanced capabilities, intelligent algorithms can be used with flying platforms to detect or predict network outages and proactively deploy flying base stations as needed, e.g., reinforcement learning for model free learning about outages in various environments. Furthermore, intelligent algorithms can be used to predict the trajectory for flying base stations to quickly create pop-up networks, e.g., deep learning techniques for obstacles detection. The efficient and most advanced design of aerial NTNs will fulfil the ultimate goal of providing anything, anytime and anywhere connectivity.
The Role of AI/ML in Aerial NTNs
Following are two comprehensive scenarios where flying platforms are expected to be integrated into future networks and where AI/ML algorithms can play a significant role toward network densification:
- Deployment configuration 1: Flying platforms are expected to complement the conventional cellular networks to further enhance the wireless capacity, expand the coverage and improve the network reliability for temporary events: i) where there is a high density of mobile users; ii) small cells in a limited/hard to reach area; iii) in a remote region where infrastructure is not available and expensive to deploy, e.g., temporary hospitals built in the UK [28], [29]. The role of AI/ML: intelligent algorithms can be used with flying platforms to detect or predict network outages and deploy flying base stations, e.g., reinforcement learning for model free learning about outages in various environments.
- Deployment configuration 2: Flying platforms can be deployed for unexpected scenarios, such as in emergency situations to support disaster relief activities and to enable communications when conventional cellular networks are either damaged or congested. In addition, owing to their mobility, flying platforms are expected to deploy quickly and efficiently to support cellular networks, enhance network quality-of-service (QoS) and improve network resilience under emergency scenarios [18]. The role of AI/ML: intelligent algorithms can be used to predict the trajectory for flying base stations to build the pop-up networks in little time, e.g., deep learning techniques for obstacles detection.
RIS-assisted NTNs
The research direction on RIS is aiming towards a reconfigurable network, which is equally valid for the above-mentioned potential and challenges of NTN technology. RIS has attracted wide attention while tending towards 5G Advanced and 6G communications [30], [31], and in joint radar-communication systems [32]. RIS technology consists of passive devices which leverage smart radio surfaces with large number of small metamaterial elements based on a programmable structure that can be used to control the propagation of electromagnetic (EM) waves. The reflection of EM waves makes RIS highly compatible with the transceiver antenna setup which can provide high capacity and coverage while benefitting from its energy-efficient characteristics.
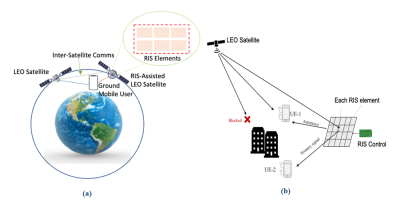
In terms of RIS-assisted NTN, for instance, RIS with multiple reflecting elements provides an alternative path between satellite and ground user when strong blockage is present on the line-of-sight (LOS) link [33]. Furthermore, RIS can be integrated with NTN entities over a wide range of available spectrum from sub-6 GHz to mmWave through to THz. Fig. 2 presents the scenarios of RIS-assisted LEO satellites, such as in Fig. 2 (a) RIS is embedded on the LEO satellite itself, whereas in Fig. 2 (b), the RIS technology is placed on a terrestrial object (e.g., building) while showing direct links with mobile users or reflecting signals via terrestrial objects. In this research direction, RIS-assisted NTNs can easily improve the reachability where macro-diversity gain comes naturally via multi-path co-phasing. Overall, the advanced technologies in LEO satellites, UAVs and other NTN entities are providing efficient, automated and flexible approaches for a complete ecosystem of NTN, having the potential to be standardized into 6G systems and networks.
In conclusion, all deployment configurations and use cases of NTN technology such as NTNs with UAVs, LEO satellites, AI/ML assisted flying platforms, reconfigurable NTNs with RIS, etc., will tend to be key technology enablers in 5G Advanced and certainly in 6G-envisioned communication systems and networks. These recent trends and benefits of implementing NTNs indicate an exponential trajectory in terms of the growth for our future wireless communication standards, resulting in a truly hyper-connected world with the type of global connectivity that can continue to close the digital divide that exists today. Along with this, the ability to quickly address disaster situations caused by humans or nature makes these networks invaluable. Many more applications will appear as industry and academia refine the capabilities of 3D networks; the “sky” will be indeed the limit.
References
- Cisco Annual Internet Report 2018-23 White Paper, Mar. 2020. [Available: https://www.cisco.com/c/en/us/solutions/collateral/executive-perspectives/annual-internet-report/white-paper-c11-741490.html]
- Ericsson Mobility Report 2022, pp. 1-40, June 2022. [Available: https://www.ericsson.com/49d3a0/assets/local/reports-papers/mobility-report/documents/2022/ericsson-mobility-report-june-2022.pdf]
- 5G Americas White Paper: Mobile Communications Towards 2030, pp. 1-56, Nov. 2021. [Available: https://www.5gamericas.org/wp-content/uploads/2021/11/Mob-Comm-Towards-2030-WP.pdf]
- 5G Americas White Paper: 5G & Non-Terrestrial Networks, pp. 1-35, Feb. 2022. [Available: https://www.5gamericas.org/wp-content/uploads/2022/01/5G-Non-Terrestrial-Networks-2022-WP-Id.pdf]
- X. Lin, S. Rommer, S. Euler, E. A. Yavuz and R. S. Karlsson, "5G from Space: An Overview of 3GPP Non-Terrestrial Networks," IEEE Communications Standards Magazine, vol. 5, no. 4, pp. 147-153, Dec. 2021.
- M. Giordani and M. Zorzi, “Non-Terrestrial Communication in the 6G Era: Challenges and Opportunities,” IEEE Network, vol. 35, no. 2, Mar./Apr. 2021, pp. 244-51.
- F. Rinaldi et al., “Non-Terrestrial Networks in 5G & Beyond: A Survey,” IEEE Access, vol. 8, pp. 165,178-200, Sept. 2020.
- O. Kodheli et al., “Satellite Communications in the New Space Era: A Survey and Future Challenges,” IEEE Communications Surveys & Tutorials, vol. 23, no. 1, pp. 70-109, 2021.
- H. Jafarkhani, “Taking to the Air to Help on the Ground: How UAVs Can Help Fight Widfires,” IEEE ComSoc Technology News (CTN), Oct. 2022. [Available: https://www.comsoc.org/publications/ctn/taking-air-help-ground-how-uavs-can-help-fight-wildfires]
- S. Narayana and R. V. Prasad, “Space, the Final “Communications” Frontier?,” IEEE ComSoc Technology News (CTN), Oct. 2022.[Available: https://www.comsoc.org/publications/ctn/space-final-communications-frontier]
- 5GPPP: Smart Networks in the Context of NGI, Sept. 2020. [Available: https://5g-ppp.eu/roadmaps/]
- ITU-R: IMT Vision-Framework and Overall Objectives of the Future Deployment of IMT for 2020 & Beyond. [Available: https://www.itu.int/dms_pubrec/itu-r/rec/m/R-REC-M.2083-0-201509-I!!PDF-E.pdf]
- “Technical specification group radio access network; study on new radio (NR) to support non terrestrial networks; (release 15), version 15.2.0,” 3GPP, Sophia Antipolis, France, Rep. 38.811, Sept. 2019.
- “Technical specification group radio access network; solutions for NR to support non-terrestrial networks; (release 16), version 0.9.0,” 3GPP, Sophia Antipolis, France, Rep. 38.821, Sept. 2019.
- 3GPP: 5G in Release 17—Strong Radio Evolution. [Available: https://www.3gpp.org/news-events/2098-5gin-release-17-%E2%80%93-strong-radio-evolution]
- ETSI ISG on RIS Activity Report 2021. [Available: https://www.etsi.org/committee-activity/activity-report-ris].
- J.-H. Lee, J. Park, M. Bennis and Y. C. Ko, “Integrating LEO Satellite and UAV Relaying via Reinforcement Learning for Non-Terrestrial Networks,” IEEE Global Communications Conference (Globecom), pp. 1-6, 2020.
- H. Ahmadi, K. Katzis, and M. Z. Shakir, “A novel airborne self-organising architecture for 5G+ networks,” IEEE VTC-Fall, Sept. 2017.
- “Technical specification group radio access network; study on enhanced LTE support for aerial vehicles (release 15),” 3GPP Technical Report 36.777, Tech. Rep., Dec. 2017.
- “New SID on enhanced support for aerial vehicles, 3GPP RP-170779 RAN#75,” NTT DOCOMO Inc., Ericsson, Tech. Rep., Dec. 2017.
- B. Li, Z. Fei and Y. Zhang, "UAV Communications for 5G and Beyond: Recent Advances and Future Trends," IEEE Internet of Things Journal, vol. 6, no. 2, pp. 2241-2263, Apr. 2019.
- W. Belaoura, K. Ghanem, M. Z. Shakir and M. O. Hasna, "Performance and User Association Optimization for UAV Relay-Assisted mm-Wave Massive MIMO Systems," IEEE Access, vol. 10, pp. 49611-49624, May 2022.
- H. Zhang, R. Liu, A. Kaushik and X. Gao, "Satellite Edge Computing with Collaborative Computation Offloading: An Intelligent Deep Deterministic Policy Gradient Approach," IEEE Internet of Things Journal, In Press, Dec. 2022.
- X. Gao, R. Liu and A. Kaushik, "Service Chaining Placement Based on Satellite Mission Planning in Ground Station Networks," IEEE Transactions on Network and Service Management, vol. 18, no. 3, pp. 3049-3063, Sept. 2021.
- X. Gao, R. Liu, A. Kaushik and H. Zhang, "Dynamic Resource Allocation for Virtual Network Function Placement in Satellite Edge Clouds," IEEE Transactions on Network Science & Engineering, vol. 9, no. 4, pp. 2252-2265, Aug. 2022.
- X. Gao, R. Liu and A. Kaushik, "Virtual Network Function Placement in Satellite Edge Computing with a Potential Game Approach," IEEE Transactions on Network and Service Management, vol. 19, no. 2, pp. 1243-1259, June 2022.
- M. Toka and W. Shin, "Outage Performance of Cooperative MISO-NOMA Based Satellite-Terrestrial Networks," 2022 IEEE Wireless Communications and Networking Conference (WCNC), pp. 155-159, 2022.
- M. Alzenad, M. Z. Shakir, H. Yanikomeroglu, and M.-S. Alouini, “FSO-based vertical backhaul/fronthaul framework for 5G+ wireless networks,” IEEE Communications Magazine, vol. 56, no. 1, pp. 218-224, Jan. 2018.
- M. Mozaffari, W. Saad, M. Bennis, and M. Debbah, “Efficient deployment of multiple unmanned aerial vehicles for optimal wireless coverage,” IEEE Communications Letters, vol. 20, no. 8, pp. 1647-1650, Aug. 2016.
- M. Di Renzo et al., “Communication models for reconfigurable intelligent surfaces: From surface electromagnetics to wireless networks optimization," Proceedings of the IEEE, vol. 110, no. 9, pp. 1164-1209, Sept. 2022.
- Q. Cheng et al., “Reconfigurable intelligent surfaces: Simplified-architecture transmitters—from theory to implementations," Proceedings of the IEEE, vol. 110, no. 9, pp. 1266-1289, Sept. 2022.
- Y. Li and A. Petropulu, “Dual-Function Radar-Communication System Aided by Intelligent Reflecting Surfaces," arXiv:2204.04721, Apr. 2022.
- J. Lee, W. Shin and J. Lee, "Performance Analysis of IRS-Assisted LEO Satellite Communication Systems," 2021 International Conference on Information and Communication Technology Convergence (ICTC), 2021.
Statements and opinions given in a work published by the IEEE or the IEEE Communications Society are the expressions of the author(s). Responsibility for the content of published articles rests upon the authors(s), not IEEE nor the IEEE Communications Society.