Andrea Alù, Einstein Professor of Physics, CUNY Graduate Center, USA
Published: 19 Oct 2020

CTN Issue: October 2020
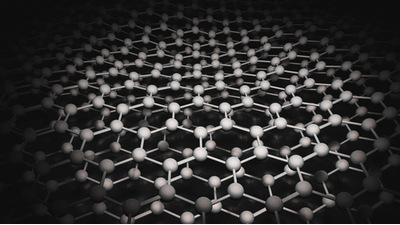
A note from the editor:
Much of how we look at communications theory today is based on the assumption that the communications signal rides on top of a nice regular sinusoid that is easily removed to provide a linear complex baseband equivalent. But as we run out of good ideas to improve current wireless modem technology, the quest for new signal processing has taken us, like ant man, into not the quantum, but the sub lambda world of meta materials. Andrea, our guide to this strange landscape where refraction and reciprocity amongst other basic physical properties, simply refuse to behave themselves, has been a leader in this field for many years now and provides some fascinating insights into what is, and may be, possible for the future of communications. Your comments as always are most welcome.
Alan Gatherer, CTN Editor-in-Chief
Metamaterials for Next-Generation Communication Systems
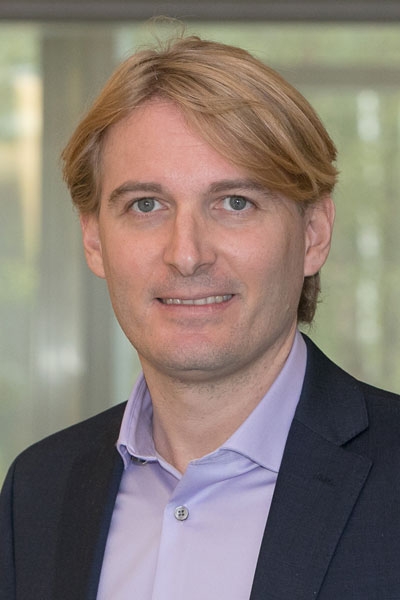
Engineered materials and metamaterials have been evolving over the past few years from an intriguing platform for physics-based exotic wave manipulation to an established technology with enormous potential for a plethora of application areas. In this brief note, I discuss recent advances in the area of metamaterials, and of their planarized version known as metasurfaces, with the goal of highlighting their potential impact for communications and wireless technology. While metamaterials have been thriving across a broad range of scientific communities, including physics, electrical engineering, material science, chemistry and mathematics, the connection with the communications society has been lagging, and the goal of this note is to provide a bridge to stimulate and spur interactions and cross-pollination between these areas to motivate new metamaterial concepts for next-generation communication systems.
1. Recent Progress in Metamaterials and Metasurfaces
Explorations of the opportunities offered by metamaterials to manipulate electromagnetic waves using enhanced and controlled wave-matter interactions started in the early 2000s, causing a revolution in material science and engineering. Metamaterials [1] are artificial materials that can be treated like homogeneous bulk media, but that offer electromagnetic properties not available in nature. The early examples of these unusual features have been based on highly unusual physical properties, such as engineering a negative or zero index of refraction, inducing anomalous reflection at an interface, or hiding an object by suppressing its scattering. In recent years, however, thanks to advances in the theoretical understanding of the opportunities and fundamental limitations of this material platform and to exciting progress in nanofabrication, metamaterials technology has matured into a solid platform to enable exciting functionalities for a variety of applications of broad interest to society.
An example that features well the opportunities offered by metamaterials and metasurfaces is featured in Fig. 1, which shows a patterned surface engineered to implement a microwave reflective lens with large numerical aperture [2]. By patterning the surface with subwavelength tailored elements, it is possible to control with great flexibility and large efficiency the local wave interactions with the surface, offering an efficient platform for wave control in antenna systems and wireless communications. By tailoring the spatial distribution of these subwavelength elements, it is possible to engineer the reflected and/or transmitted wavefront emerging from the metasurface, enabling extreme wave manipulation over an ultrathin platform, ideally suited for wireless communications.
Interestingly, this concept is not limited to local manipulations of the electromagnetic wavefront in the spatial domain, but they can be extended to broadly control the spectral content, both in space and in time, of the impinging signals. An extreme example is the possibility to engineer metasurfaces that can process the impinging signals in the analog domain [3], in order to impart at the speed of light mathematical operations of choice on the impinging wavefront. While so far the application of these concepts have been concentrated in the optical domain, for instance for edge detection and object identification, several opportunities may be envisioned to apply these concepts also to communication systems, so that a computing metasurface may offload some of the signal processing aspects typically tackled in the digital domain through slow and energy intensive processes, but now imparted by a passive metasurface analogically, at very fast speeds and with essentially zero energy costs.
These concepts, among many others, have been thriving in the metamaterials community, with implications not limited to the radio-frequency spectrum, but also to manipulate THz, mid-infrared and light waves, as well as sound and mechanical waves.
![Figure 1: From [2]. A metalens with large numerical aperture that can efficiently focus an impinging wave into a diffraction-limited spot close to the surface, within a deeply subwavelength profile.](/sites/default/files/styles/400wide/public/images/2020-2020-10/figure1.jpg?itok=nOMDulcc)
2. Nonreciprocal and Active Metamaterials
A particularly active area of recent research progress in the context of metamaterials and metasurfaces, with important opportunities for the next generation of communications technology, is offered by the possibility of breaking reciprocity in compact, ultrathin devices. Reciprocity is the symmetry relations that governs propagation of waves between two points in space. If a radio-wave signal can travel from point A to B, it can travel back with similar properties from B to A. Breaking this symmetry is particularly useful for communication systems: it enables one-way transmission of signals that avoids self-interference and protects sources, and it can be used for implementing full-duplex communications with significant advantages in terms of spectrum efficiency.
Since reciprocity is rooted in the time-reversal symmetry of Maxwell’s equations, it can be broken using a dc bias to the involved materials, imparting spatio-temporal modulation of the channel properties, or through nonlinearities combined with geometrical asymmetries [4]. The most common way of breaking reciprocity is through a dc magnetic bias applied to ferrites or garnets that support magneto-optical phenomena. While this approach is effective in realizing isolators and circulators commonly used in radar and antenna systems, the resulting devices are bulky, expensive and heavy, not amenable for integration. This is why there has been a recent concerted effort to employ metamaterials to realize magnet-free devices that may lead to the next generation of compact, integrated nonreciprocal technologies, with performance metrics comparable and even superior to their magnetized counterparts, but at a fraction of the cost, weight and form factor [5]. Fig. 2 shows recent examples of printed circuits and integrated circuit technologies that implement isolators, circulators and nonreciprocal antennas, opening new opportunities for a broad range of applications, from wireless communications to automotive technologies and quantum computing, which cannot afford magnetic approaches to breaking reciprocity, but that would largely benefit from the implementation of these functionalities in a compact, integrable form factor [4-5].
![Figure 2: Printed and integrated circuits featuring compact magnet-free isolators and circulators recently built in our group to implement nonreciprocal functionalities compatible with CMOS technology (see [4] for a recent review)](/sites/default/files/styles/400wide/public/images/2020-2020-10/figure2.png?itok=-RMTN1z5)
These technologies exploit modulation and switching schemes to implement tailored spatio-temporal modulation patterns that replace magneto-optical phenomena in a compact device for the purpose of breaking reciprocity, opening truly exciting directions for various technologies. Interestingly, these compact nonreciprocal elements can then also be employed as building blocks of new metamaterials and metasurfaces, with yet even more unusual wave manipulation properties. In metasurfaces, this technology can manipulate the impinging wavefront in nonreciprocal waves, implementing free-space isolation and nonreciprocal radiation [6], while for guided waves arrays of magnet-free subwavelength nonreciprocal elements can realize the electromagnetic version of topological insulators, which provide unusual robustness to disorder, broad bandwidths, and robust multiplexing opportunities [7].
Temporal modulations imparted over metamaterials offer also other interesting opportunities beyond breaking reciprocity. Parametric phenomena enable gain that may pump the impinging signals and compensate insertion loss over a low-noise platform. In addition, suitably tailored spatio-temporal modulations can overcome the limitations of passive, linear, time-invariant devices, with important implications for communications technology. As examples, our group has recently shown that time-modulated antennas may overcome the Chu limit, which conventionally enforces a stringent trade-off between size and bandwidth of an electrically small antenna [8], and that time modulated circuit networks can overcome the trade-off between delay and bandwidth [9], ideally suited for true time delay applications. In this context, the N-path filter platform [10], formed by CMOS compatible time-commutated networks of integrated switches and capacitors, provides an ideal technology to enable many of these principles, and it is an outstanding and mostly untapped opportunity to integrate these concepts into metamaterials and metasurfaces.
The next avenue in this context consists in the possibility of leveraging time modulations to impart reprogrammability and reconfigurability on the fly, with also the option of active feedback and control, based on which active and time-varying metamaterials can reprogram their own function in response to external stimuli or changes in the environment.
3. Conclusions
The field of metamaterials has been progressing at a fast pace in the past years, showing that the conventional limitations of modern technology can be pushed forward and overcome using advanced electromagnetic concepts and nanofabrication techniques. It is the right moment for exploring these features in the context of next-generation communication systems. We may envision smart metasurfaces that can process the impinging signals, focus, steer and route them in desired directions and self-reconfigure their operations on the fly as the environment changes, all with strong robustness to noise and disorder. The implementation of these surfaces can be achieved with standard lithographic or 3D printing techniques. Still of some challenge remains the implementation of reconfigurable and time varying elements in metasurfaces, which may require complex control networks and advanced circuit layouts, especially in the case of fast modulation speeds. Discussions and collaborations between the communications and metamaterial communities may be extremely fruitful to push forward these avenues and converge on a technology platform that holds the potential to revolutionize several application areas for next-generation communication systems.
Acknowledgements
Our work on metamaterials has been funded over the years by the Department of Defense, the National Science Foundation, the Simons Foundation, among several other funding agencies, foundations and companies.
References
- N. Engheta, R. W. Ziolkowski, “Metamaterials: physics and engineering explorations,” John Wiley & Sons (2006)
- M. Kang, Y. Radi, D. Farfan, and A. Alù, “Efficient Focusing with Large Numerical Aperture Using a Hybrid Metalens,” Physical Review Applied, Vol. 13, 04416 (9 pages), April 7, 2020
- H. Kwon, D. L. Sounas, A. Cordaro, A. Polman, and A. Alù, “Nonlocal Metasurfaces for Optical Signal Processing,” Physical Review Letters, Vol. 121, No. 17, 173004 (6 pages), October 24, 2018
- A. Kord, D. Sounas, and A. Alù, “Magnet-Free Microwave Nonreciprocity,” Proceedings of IEEE, in press (published online on July 17, 2020)
- D. Sounas, and A. Alù, “Non-Reciprocal Photonics Based on Time Modulation,” Nature Photonics, Vol. 11, No. 12, pp. 774-783, November 30, 2017
- Y. Radi, and A. Alù., “Nonreciprocal Wavefront Manipulation in Synthetically Moving Metagratings,” Photonics, Special Issue for Metamaterials 2019, Vol. 7, No. 2, 28 (7 pages), April 18, 2020
- R. Fleury, A. Khanikaev, and A. Alù, “Floquet Topological Insulators for Sound,” Nature Communications, Vol. 7, No. 11744 (11 pages), June 17, 2016
- H. Li, A. Mekawy, and A. Alù, “Beyond Chu’s Limit with Floquet Impedance Matching,” Physical Review Letters, Vol. 123, No. 16, 164102 (6 pages), October 16, 2019
- M. Tymchenko, D. L. Sounas, A. Nagulu, H. Krishaswamy, and A. Alù, “Quasielectrostatic Wave Propagation Beyond the Delay-Bandwidth Limit in Switched Networks,” Physical Review X, Vol. 9, No. 3, 031015 (16 pages), July 31, 2019
- N. Reiskarimian, and H. Krishnaswamy, “Magnetic-free non-reciprocity based on staggered commutation,” Nature Communications, Vol. 7, No. 11217, 2016
Statements and opinions given in a work published by the IEEE or the IEEE Communications Society are the expressions of the author(s). Responsibility for the content of published articles rests upon the authors(s), not IEEE nor the IEEE Communications Society.