Josep M. Jornet, Northeastern University, USA
Published: 22 Nov 2019

CTN Issue: November 2019
A note from the editor:
If there is a trend in wireless communications, it is the unrelenting increase in signal bandwidths. For decades, bandwidths have increased within a restricted –and increasingly congested– frequency range in the microwave realm, between 1 and 6 GHz, where radio propagation is favorable. Higher frequency bands were perceived as inhospitable because of the harsh propagation conditions and the prohibitively expensive hardware. In recent years, though, research has broken through the mmWave frontier, and early 5G deployments are underway with components in the 30-GHz bands and above. Transmission ranges have become short enough to be compatible with mmWave propagation, which in turn has revealed itself somewhat more benign than anticipated.
With the gates of higher frequencies open, perceptions have changed and now it seems that the sky is the limit, to the point that researchers are already looking into the sub-THz (100-300 GHz) and full THz realms, where many GHz of idle bandwidth await. This is precisely the subject of this month’s article by one of the leading investigators on the matter. Enjoy.
- Angel Lozano, Editor
Terahertz Communications: The Quest for Spectrum
Josep M. Jornet
Associate Professor
Department of Electrical and Computer Engineering
Northeastern University, Boston, MA, USA
jmjornet@northeastern.edu
Wireless data traffic has surged in recent years due to changes in the way society creates, shares, and consumes information. This surge calls for ever faster, more ubiquitous wireless communication networks. Following the current trend, wireless Terabit-per-second (Tb/s) links are expected to become a reality in less than five years. How are we going to achieve that feat? Current wireless systems are mainly employing spectrum under 95 GHz [1], where bandwidth is limited and thus all efforts are on increasing the spectral efficiency. At the other end of the spectrum (above tens of THz), optical wireless communication systems at infrared, visible or even ultra-violet frequencies are being developed with the promise of virtually unlimited bandwidth (at the cost of less advantageous wireless propagation). However, if bandwidth is the driver, why would someone ignore the almost 10 THz of consecutive bandwidth, right above the microwave and millimeter waves and below the far infrared?
The THz Band: No Man’s Land…Or Is It?
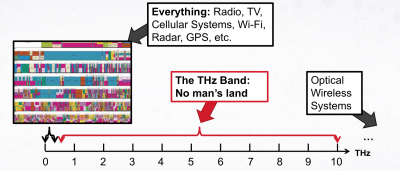
The THz band (from 100 GHz to 10 THz, also known as the sub-millimeter wave spectrum, Tremendously High Frequencies or Extreme Far Infrared) is still one of the least explored frequency bands for communications…but this is certainly not the case for the remote sensing community. Beyond the unique electromagnetic signature of different materials at THz frequencies (which has motivated the use of this frequency band for nondestructive imaging and testing), what many do not know is that “one-half of the total luminosity and 98% of the photons emitted since the Big Bang fall into the submillimeter and far-IR” [2]. This is the reason that major space-focused research groups and agencies, such as NASA and ESA, have been developing THz sensors for decades and, as a result, while you read this article, there are satellites with THz radios orbiting the Earth.
But…What Happens with THz Technology?
The truth is that, for many years, this band has also been known as the THz gap, mainly because of the lack of compact, energy efficient device technologies able to generate, modulate, detect and demodulate THz signals at room temperature. Traditionally, the THz band seemed to be “too high” for the RF electronics community and “too low” for photonics engineering. However, what used to be a problem has now become an opportunity, as both communities are (often jointly) developing new solutions for practical THz transceivers and antennas [3], with increased transmission power, modulation bandwidth, and receiver sensitivity. Moreover, by leveraging the properties of (relatively) new two-dimensional materials such as graphene and novel physics such as plasmonics, which lie at the intersection of electronics and photonics, new on-chip plasmonic sources & detectors, modulators & demodulators, and antennas and (reflect) antenna arrays that naturally operate at THz frequencies are being conceived [4].
But…The THz Channel Is Horrible, Isn’t It?
Another aspect that has traditionally prevented the communications community to enter this frequency band has been the perception that the absorption by water vapor molecules renders the THz spectrum unusable for practical applications. Indeed, there is molecular absorption, yet not as a constant loss across frequencies, but rather as a collection of high attenuation peaks or absorption lines that define multiple transmission windows [5]. While the width of such windows depends on factors such as the humidity and the transmission range, each can easily exceed 100 GHz, enough to fit all the wireless communication services currently under 95 GHz. Many of the early works on THz channel experimental characterization were focused around the 200-300 GHz window [6], but the state of the art is now pushing into the first absorption-defined window above 1 THz [7]. Besides absorption, there are other aspects, including the fact that THz signals cannot propagate through and suffer from reflections and diffraction. All of this makes THz propagation challenging and poses as major challenge the increase in the communication range [8]. Still, THz signals propagate much better than optical signals while offering comparable bandwidths.
So, How Can We Make the Most Out of It?
New communication and networking strategies that take into account the capabilities of THz transceivers and antennas, and the peculiarities of the THz channel, are needed to conquer the THz band [9]. For example, at the physical layer, while traditional modulation schemes can be utilized, these cannot make the most out of the unique distance-dependent transmission bandwidth of the THz band, which can be seen as a problem, but also as an opportunity for physics-enabled spatial multiplexing [10]. Similarly, there are many challenges associated to the synchronization in time, frequency, and phase of Tb/s signals transmitted with relatively low power over ultrabroad bands. Moreover, in many cases, traditional solutions based on digital signal processing cannot be employed because of the lack of digital-to-analog and analog-to-digital converters operating at the minimum required sampling frequency defined by Nyquist. Perhaps, in addition to speeding up digital electronics and massively parallelizing (relatively) narrow channels, a second chance should be given to analog electronics, potentially guided by brain-inspired and artificial-intelligence-based solutions. Obviously, all this needs to happen synergistically with the development of up-to-date and supportive spectrum policies and legislations [11], as well as standardization efforts [12].
Will All This Be Worth It?
We started this article talking about the need for wireless Tb/s links and, yes, THz communication will contribute to enabling interesting and needed applications such as immersive virtual reality (for remote medicine and precision surgery), ultrabroadband connectivity for rural areas (where fiber-optical deployments are not possible), or even a Terabit backhaul in the sky (above the atmosphere, where there is no absorption). Perhaps more interestingly, by leveraging the very small size of THz transceivers and antennas (sub-millimetric and, when leveraging plasmonic physics, sub-micrometric), THz communication opens the door to transformative applications that include new nano-bio-sensing wearable and implantable devices that interact with our body at the actual biological scale--the diameter of a red-blood cell is similarly tens of micrometers--wireless brain machine interfaces able to interact with neurons as opposed to brain regions, and others that we just do not know yet.
References
- US Federal Communications Commission, “FCC Online Table of Frequency Allocations,” retrieved November 2, 2019.
- P. Siegel, "Terahertz Technology,” IEEE Transactions on Microwave Theory and Techniques, vol. 50, no. 3, pp. 910-928, 2002.
- K. Sengupta, T. Nagatsuma, and D. Mittleman, “Terahertz integrated electronic and hybrid electronic–photonic systems,” Nature Electronics, vol. 1, no. 12, p. 622, 2018.
- J. M. Jornet and I. F. Akyildiz, “Graphene-based plasmonic nano-transceiver for terahertz band communication,” in Proc. of the 8th IEEE European Conference on Antennas and Propagation (EuCAP), pp. 492–496, 2014. U.S. Patent No. 9,397,758, July 19, 2016.
- J. M. Jornet and I. F. Akyildiz, “Channel Modeling and Capacity Analysis for Electromagnetic Wireless Nanonetworks in the Terahertz Band,” IEEE Transactions on Wireless Communications, vol. 10, no. 10, pp. 3211-3221, October 2011.
- C. Jastrow, K. Munter, R. Piesiewicz, T. Kurner, M. Koch, and T. Kleine-Ostmann, “300 GHz transmission system,” IET Electronics Letters, vol. 44, no. 3, pp. 213–214, 2008.
- P. Sen and J. M. Jornet, "Experimental Demonstration of Ultra-broadband Wireless Communications at True Terahertz Frequencies," 2019 IEEE 20th International Workshop on Signal Processing Advances in Wireless Communications (SPAWC), Cannes, France, 2019, pp. 1-
- I. F. Akyildiz, C. Han, and S. Nie, “Combating the distance problem in the millimeter wave and terahertz frequency bands,” IEEE Communications Magazine, vol. 56, no. 6, pp. 102–108, 2018.
- I. F. Akyildiz, J. M. Jornet and C. Han, “Terahertz Band: Next Frontier for Wireless Communications,” Physical Communication (Elsevier), vol. 12, pp. 16-32, Feb 2014.
- Z. Hossain and J. M. Jornet, “Hierarchical Bandwidth Modulation for Ultra-broadband Terahertz Communications,” in Proc. of the IEEE International Conference in Communications (ICC), Shanghai, China, May 2019.
- US Federal Communications Commission, “Spectrum Horizons,” ET Docket No. 18-21, RM-11795, March 15, 2019.
- IEEE 802.15 Wireless Personal Area Networks – Terahertz Interest Group, Online: http://www.ieee802.org/15/pub/TG3d/index_IGthz.html.
Statements and opinions given in a work published by the IEEE or the IEEE Communications Society are the expressions of the author(s). Responsibility for the content of published articles rests upon the authors(s), not IEEE nor the IEEE Communications Society.