Marcus Brunner, ETSI ISG F5G Liaison Officer and Chief Expert Huawei Technologies Switzerland, Zurich
Published: 24 Mar 2023

CTN Issue: March 2023
A note from the editor:
We spend most of our lives listening to TV commercials about 5G and all the wonderful things it can do for you, and of course we know that 5G relates to wireless communications. For those of us that are in the muck of 3GPP standards, we tend to lose sight of other technologies that are fundamental to the success of wireless communications. Enter F5G or Fifth Generation Fixed Networks. Given all the hype about ChatGPT and given the lack of understanding that I have about optical networking, I decided to ask Bing Chat to write a short intro to an article about F5G, and this is what it came up with: “F5G is the latest advancement in fixed network telecommunication technology. It serves for the optical bandwidth access of fixed networks through wiring and is different from 5G, which is the fifth generation of mobile telecommunication that mainly serves for the access of mobile terminal devices like mobile phones. F5G will open new opportunities by comprehensively applying fiber technology to various scenarios and expanding the reach of fiber to everything, everywhere. This will benefit all industry verticals such as telecom, education, healthcare, finance, energy, transportation and manufacturing. In this article, we will explore what F5G is and how it can improve our daily lives.” Interesting, it even told us what we can expect from the article. So, this month Marcus Brunner was kind enough to give us a bird’s eye overview of optical networks and in particular F5G Advanced. Enjoy it.
Miguel Dajer, CTN Editor-in-Chief
The Evolution of Broadband to F5G Advanced
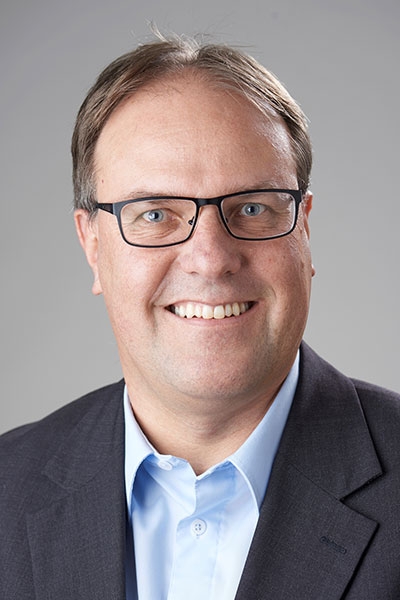
Marcus Brunner
ETSI ISG F5G Liaison Officer and Chief Expert
Background
One of the overarching goals of the ETSI Industry Specification Group (ISG) F5G on Fifth Generation Fixed Network is to establish a regular rhythm of evolution for the fixed network. This technology enhancement corresponds to the series of generations defined for the wireless network. So far, the ETSI ISG F5G has published technical specifications for the F5G network on use cases, generations definition of fiber networks, architecture, and more [1]. The evolution towards F5G Advanced has started and the group will work on those F5G standards. Also note that various global research activities started towards F6G. See figure 1 for an illustration of the generational roadmap from F5G to F6G.
![Figure 1: Illustration of the F5G Advanced roadmap. Source ETSI [2].](/sites/default/files/styles/400wide/public/images/2023-2023-03/ctn-march-2023-figure1.png?itok=YA9OMq-S)
Six Dimensions of the F5G Advanced Network Capabilities
Nine key applications or industry trends are identified as key drivers for F5G Advanced. These can be grouped into two categories: those that are oriented towards services and applications, and those that are directed towards network transformation and infrastructure improvements. Ultra-high-definition immersive experience services could put many new requirements on the network. Enterprises will continue their digitization and cloudification, and this is a huge opportunity for fixed networks. Industrial applications of fiber networks offer completely new markets for providers as well as add value for manufacturers. The metaverse could be a driver for new infrastructure capabilities. The digitization of network operations can help to reduce operational costs and improve service agility. Optical fiber infrastructure continuing to expand, becoming nearly ubiquitous. Smart society infrastructures can improve services for all while reducing their cost. Overcoming the green challenge is critically important for the planet and the sustainability of the network. At the same time, the business environment continues to change, and this can have major impacts on the fixed network.
Given all of these drivers, the capability of the network is envisioned to increase in six main dimensions. See Figure 2 for an illustration including a set of sample applications per dimensions.
Faster: Networks will become faster, increasing bandwidth to accommodate more services.
Quicker: They will become quicker, reducing latency as much as physically possible to enable new applications, for example, industrial applications.
Wider: Networks will become “wider” by increasing the network scope and number of fiber and connection endpoints, growing fiber to everywhere and everything.
Greener: Fiber networks are already much greener compared to copper networks; these networks can also have a secondary effect in green initiatives. As an example, lowering emissions in travel as virtual experiences become more common.
Smarter: Information systems will become smarter by integrating computing power into the network at all levels and using it for applying AI technologies for smart operation and compute as a service application model.
More aware: Networks will also become more aware to help improve operations and maintenance. And in addition, the use of fiber sensing capabilities can enable or improve the services.
![Figure 2: The six dimensions of F5G Advanced. Source ETSI [2].](/sites/default/files/styles/400wide/public/images/2023-2023-03/ctn-march-2023-figure2.png?itok=dOO0OwG1)
Key Technologies for F5G Advanced
To propel the growth of the network along these six dimensions, various new technologies will be needed. The following technologies are identified as important to support the evolution of F5G Advanced networks.
Network Technologies
In the F5G architecture, there are three major divisions of networks: transport, access, and customer premises.
Transport technologies include 800G+ OTN transmission technologies, additional flexibility in the modulation rate and format, as well as other features such as constellation shaping and advanced error correction. Sub-1G OTN provides ubiquitous optical connection services with premium quality. Optical Cross Connects enable all optical energy efficient transmission much further to the edge of the network.
The access network technology is 50G-PON, which has enough capacity to be used in many areas and has the advantage that it is centrally scheduled, and completely deterministic in how and when each ONU transmits.
The 50G-PON access technology is defined in the ITU-T G.9804 series, where it describes a single channel system with 50 Gb/s downstream and 12.5 Gb/s, 25 Gb/s, or 50Gb/s upstream capacity. Future enhancements will include multi-channel operation. The primary technical advancement in 50G-PON is the use of DSP to resolve many of the PON and component impairments. 50G-PON has enough capacity that it becomes a viable alternative to a conventional switch-based network.
The customer premise network technologies include Fiber-to-Room (FTTR) and Wi-Fi7 for high Quality of Experience (QoE) in residential and enterprise scenarios. Wi-Fi 7 can achieve larger than 30 Gb/s peak throughput as compared with 9.6 Gb/s for its predecessor. Wi-Fi 7 also supports backward compatibility with all of the previous generations of Wi-Fi and can use the 6 GHz band for higher throughput and lower interference. It supports more MIMO streams. It also promises to have lower latency and support for time sensitive networking, and it supports multi-link operation that enables the simultaneous use of multiple RF bands.
Figure 3 shows the preliminary thinking in ETSI ISG F5G on the main features and characteristics of F5G Advanced. Note though that is preliminary and not finally decided and might change in the discussion in the community.
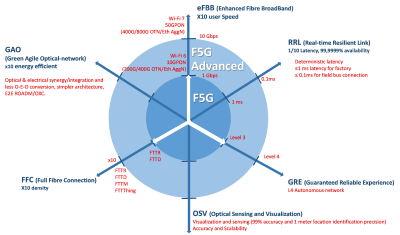
Fiber to the Thing
Fiber technologies will be advanced to support FTTRoom (FTTR), FTTMachine (FTTM), and FTTCampus/Office (FTTO). This also means the digitalization of fiber deployments for operating it at scale and with minimal manual work. Also, quick and easy installation of fibers is required since it might need to be done by non-experts. For Fiber-to-the-Machine, the use of carrier-grade reliability, security, and performance technologies are required and eventually need to be enhanced for certain use cases.
Latency Control
Latency will be controlled using techniques such as deterministic networking (resource reservation, scheduling mechanisms) and end-to-end slicing (separation and isolation of traffic and tightly controlled traffic steering).
Latency and jitter of Wi-Fi interfaces are key bottlenecks in home/campus office scenarios. Wi-Fi 7 uses OFDMA technology, multi-user resource allocation, and multi-link coordination algorithms to implement slicing over the air interface. This effectively reduces conflicts over the Wi-Fi air interface, improving service forwarding delay and jitter, and achieving a deterministic low latency of milliseconds to meet service requirements.
The optical access network can introduce the dual-plane forwarding architecture that adds the TDM forwarding plane to the existing packet forwarding plane. The traffic that is handled by the TDM-plane can be easily handed over to an OTN system, reducing latency even further. Jitter compensation mechanisms, single-frame multi-burst technology, independent registration channel technology, and collaborative DBA (Dynamic Bandwidth Allocation) technology are introduced to implement microsecond-level low-latency forwarding and microsecond-level service jitter.
On an optical transport network, the fiber latency per kilometer is 5 µs. The fiber latency is a major contribution to the end-to-end link delay. Selecting the optimal (shortest) physical path based on the network service direction or traffic ownership can greatly reduce the latency. In network planning, multi-path mesh or mesh structure are considered. The network architecture supports all-optical grooming and small-granularity electrical grooming capabilities, improving the low-latency grooming capability of the network to meet service requirements. The next-generation OTN container technology for small-granularity bearer (Sub-1G OTN) will greatly reduce the latency of single-node devices by reducing multiplexing layers based on virtual channel technology. Connections that meet deterministic low-latency requirements can be based on hard segregated traffic pipes.
E2E slicing ensures SLA experience over diverse Wi-Fi air interfaces, PON networks, and transport networks. By accurately sensing application SLA requirements, dynamic slice creation and on-demand resource scheduling can be implemented, and SLAs can be visible, manageable, and assured. There can be multiple types of slices, include a strict type that reserves resources unconditionally, and a permissive type that allows the reallocation of resources when not in use by the primary slice user. To achieve accurate and fast provision of E2E slicing, F5G Advanced networks need to be able to flexibly configure and combine infrastructure resources and have the ability to analyze and control E2E slicing
Energy Efficiency
Energy efficiency can be supported at the network, equipment, and application levels.
The F5G Advanced network architecture will change to be flatter and a more optical oriented network architecture removing electrical communication technologies where possible. This enables implementing "one-hop transmission of wavelengths". In combination with optical and electrical synergy networking, wavelength grooming and pass-through of intermediate nodes can effectively reduce power consumption of intermediate nodes caused by opto-electrical regeneration.
Also does the F5G Advanced architecture include energy aware switching and routing. In order to efficiently utilise energy, low loaded light-paths can be deactivated, and new light-paths are activated in case of traffic congestion. This can be done based on thresholds specified in the literature and dynamically modify virtual topology of the network.
Power consumption decreases along with technology advancement, since larger bandwidth with equal or lower energy usage are expected. The equipment will include power save modes and more efficient technologies such as Co-Packaged Optics (CPO).
Distributed Computing
Computing networks are by their nature distributed, and they change the usage model from resource-based to task-based. To ensuring high quality, it is necessary to enhance the system capabilities in terms of elasticity, flexibility, and intelligent scheduling to provide network resources on demand. Network resources can be obtained upon use and released when no longer needed (the cloud model) and needed for any sort of cloud access.
Technologies for high quality computing integrated into the network include elastic joint network and compute resource scaling and latency-aware process dispatching. High-quality computing is a way to have guaranteed computing services over the network, which means the access to the processing resource and the guaranteed computing resource. Basically, the end-to-end network path from customer device to the processing device can be diverse and could possibly include in-premise, access, aggregation, core, and data-center networks, and is required to be high-quality. All the network elements need to support resource allocation for guaranteed services. The processing device needs to guarantee high quality service guarantees. Computing power can be integrated into the network nodes or can be independent.
For latency-oriented services, the service provider’s workload placement system can choose to place workload at the edge of the network in a distance enabling to guaranteed latency and when enough resources are available.
Autonomous Network Management Technologies
To implement autonomous networking, the Telecommunication Management Forum (TMF) proposes a four-layer three-closed-loop autonomous network (AN) architecture. Through cooperation and collaboration between the network element layer, network layer, service layer, and business layer, the TMF implements closed-loop management of resources, services, and businesses. It provides consumers and enterprises with a high-quality network that enables zero wait services, zero touch service optimization, and zero trouble network maintenance. It implements network automaticity through core technologies such as service intent-driven, multi-dimensional experience awareness, intelligent bottleneck identification, and network auto-adaptation.
The first step is to define the various levels of autonomy in the optical networking domain such that improvements can be measured. To enable autonomous network management, techniques such as intent-based management, knowledge graph for fault management, and network information gathering are used.
Network-based Sensing
Network-based sensing systems include fiber cable digitization and detection mechanisms, distributed optical fiber sensing for measuring temperature, vibration, etc., and Wi-Fi sensing with its application on the home or enterprise installation for various applications, for example, the localization of objects or humans.
By using multiple optical signal detection technologies based on advanced passive photonics and integrated photoelectric detection devices, combining spectral signal event recognition algorithm and computational vision image recognition algorithm, the problem of fiber and passive cable resource management is effectively solved.
Mainstream optical fiber sensing technologies include distributed optical fiber vibration monitoring based on Rayleigh scattering effect, distributed optical fiber temperature measurement based on Raman scattering effect, and distributed temperature and strain monitoring based on Brillouin effect. The technology is characterized by anti-electromagnetic interference, anti-corrosion, easy integration, inherent safety, long distance, and high precision, and has been widely demonstrated in large-scale engineering projects. In recent years, narrow linewidth light sources have been gradually cost reduced and miniaturized. Channel algorithms have been optimized, and artificial intelligence has been applied to process big data generated by optical fiber sensors to implement automatic event identification.
Indoor network deployment based on Wi-Fi allows a high throughput data rate with multiple users. Such network can serve to provide Wi-Fi sensing of targets, particularly humans, but extendable to objects, animals, and environment. Currently, Wi-Fi sensing at sub-6 GHz is mainly based on measuring channel state information (CSI) which leads to low accuracy due to high wave diffractions and wide antenna beams. Because the indoor environment is complex and susceptible to interference, and the wireless signal transmission is highly dynamic, the wireless signal is not stable, which limits the accuracy of Wi-Fi sensor identification. Improvements for Wi-Fi sensing are expected.
Standardization Methodology and Expected Timeline
ETSI ISG F5G has started standardizing F5G Advanced in early stage at the time of publication of this article. The methodology applied is a top-down approach starting with the definition of the F5G Advanced generation specifying the functional and performance characteristics listed above in more detail, then gathering use cases of what an F5G Advanced network shall be able to achieve from a user or customer perspective. Based on that the technical requirements for each use case are defined and checked against available standards. The missing functions are either specified in the ETSI ISG F5G group or are liaised to the appropriate standardization groups defining a particular technology. Finally, the F5G Advanced overall end-to-end fixed network architecture is specified covering all network segment from the end-systems like machines, devices, things to the cloud traversing customer premises, access, aggregation, core network to the cloud.
The ETSI ISG F5G has adopted a release-based approach to standardization. This means also that several releases for a generation are specified. The reason is that not all features are required at the same time. Figure 4 shows the currently expected timeline, subject to the progress and decisions of the ETSI ISG F5G group.

Conclusion
The F5G generation for fixed networks is defined and currently deployed globally. Now it is time to specify F5G Advanced with improvements along various dimensions and additional features and better performance to cover a larger and more diverse set of networked applications. For the current status of the work of the ETSI ISG F5G refer to [1].
For further evolving the fixed network, new technologies are also needed beyond F5G Advanced. A first set of potential technologies has been listed in [2]. Many of those new technologies need to be researched, and the technical and commercial feasibility needs to be assessed.
References
- ETSI ISG F5G Web page including links to the reports and specifications, https://www.etsi.org/committee/f5g
- ETSI White paper No#50 Fixed 5th Generation Advanced and Beyond, September 2022, ISBN No. 979108262071
- What do You Need to Know about F5G? Damon Watson, 5 July 2022, What do You Need to Know about F5G? | V-SOL (vsolcn.com)
Statements and opinions given in a work published by the IEEE or the IEEE Communications Society are the expressions of the author(s). Responsibility for the content of published articles rests upon the authors(s), not IEEE nor the IEEE Communications Society.