Marco Di Renzo, FIEEE, French National Center for Scientific Research (CNRS), CentraleSupélec – Paris-Saclay University, Paris, France
Published: 20 Sep 2021

CTN Issue: September 2021
A note from the editor:
Reconfigurable intelligent surfaces aka RIS, can adapt or change the radio signals between the transmitters and receivers giving birth to the new concept of wireless as a service. In this article, the author Marco Di Renzo, presents the historical emergence of the RIS and highlights some future applications. He argues that recent research results confirm that effective deployment of RIS could enhance capacity, coverage and security on one side, and on other side RIS can create opportunities for future applications such as positioning and localisation by exploiting backscatter communication principles. As a new idea it does sound promising, as with any new technology RIS is not an exception when it comes to challenges. Signal Processing, implementation, deployment and cost related challenges are a few to name when controlling the behavior of the wireless communication channel.
As you can imagine many researchers around the world are working on this technology. If you would like to learn more about RIS then this article is a must read. It presents ongoing research activities including recently funded projects, so you know where to look for recent directions, research interest groups (e.g., Special Interest Group within IEEE ComSoc Wireless Communications Technical Committee) and other recent standardisation and study groups activities. As always, we look forward to your comments.
Muhammad Zeeshan Shakir, CTN Associate Editor
Reconfigurable Intelligent Surfaces for Smart Radio Environments: From Concept to Standardization?
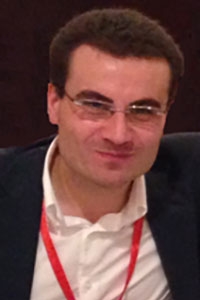
Marco Di Renzo
IEEE Fellow
We live in a world of waves, most commonly electromagnetic waves. During the last decades, we learned how to utilize many kinds of waves for transferring information data between two or more points without the need of using any electrical conductor as the medium to perform the transfer. How about controlling and creating new wave effects for communications?
In a pair of papers published in 2005 [1] and 2003 [2], we can read
“We see that if a scatterer can be chosen such that the electric and/or magnetic polarizability is changeable on demand, then a smart or controllable surface would be realized”,
and
“This could in principle enable us to realize controllable surfaces, able for instance to switch electronically between reflecting and transmitting states”,
as well as
“…are expected to have wide application to the design and analysis of antennas, reflectors, and other devices where controllable scatterers are used to form a smart surface”.
Further to this, in a paper published in 2010 [3], we can read
“This paper deals with a new approach to the control of the propagation environment in indoor scenarios using intelligent walls. The intelligent wall is a conventional wall situated inside a building, but equipped with an active frequency selective surface and sensors. The intelligent wall can be designed as a self-configuring and self-optimizing autonomous part of a collaborative infrastructure working within a high-capacity mobile radio system. The paper shows how such surfaces can be used to adjust the electromagnetic characteristics of the wall in response to changes in traffic demand, monitored using a network of sensors, thereby controlling the propagation environment inside the building”.
Tracing back the history of an emerging field of research is never a simple task and it is not the aim of this short technology note. Nevertheless, we can recognize in these short excerpts the main essence of an emerging concept to wireless system design and of an enabling technology that have been receiving major research interest during the last three years. They are usually referred to as smart or programmable radio environments as well as reconfigurable intelligent surfaces, intelligent reflecting surfaces, large intelligent surfaces, holographic surfaces, digitally controllable scatterers, and the like. In this technical note, for ease of writing, we adopt the terms smart radio environments (SREs) and reconfigurable intelligent surfaces (RISs), as they have been adopted by a recently established Industry Specification Group (ISG) within the European Telecommunications Standards Institute (ETSI), whose objective is to coordinate and streamline pre-standardization research efforts on RIS-empowered SREs across various collaborative projects towards paving the way for future standardization of the RIS technology [4].
But, let’s proceed step-by-step…
From 5G to 6G
With the current deployment of the fifth generation (5G) of telecommunication standards, it is now a critical time to identify new enabling technologies for the sixth generation (6G) of communication systems. 6G networks are expected to fulfill more stringent requirements compared to 5G systems, in terms of transmission capacity, reliability, latency, coverage, energy consumption, and connection density. Existing 5G technologies, such as millimeter-wave communications, massive multi-input multi-output systems, ultra-dense heterogeneous networks, are mainly focused on system designs at the transmitter and receiver ends, as well as on deploying additional network infrastructure with power amplification, digital signal processing capabilities, and backhaul and power grid availability. The purpose of currently available 5G technologies is mainly to cope with or to capitalize on often-unfavorable wireless propagation environments. In fact, the wireless environment is conventionally modeled as an exogenous entity that is usually not directly controlled by the network operators, but the network infrastructure and the user terminals usually adapt to it. According to this design paradigm, communication engineers usually design the transmitters, receivers, and transmission protocols based on the specific properties of the wireless channels in order to achieve the desired and target key performance indicators [5], [6], [7], [8].
![Figure 1: Illustrative diagram of a reconfigurable intelligent surface [9].](/sites/default/files/styles/400wide/public/images/2021-2021-09/ctn-202109-figure1.png?itok=xm92tZiT)
![Figure 2: Example of manufactured reconfigurable intelligent surface and corresponding phase-amplitude response (varactor-controlled) [10].](/sites/default/files/styles/400wide/public/images/2021-2021-09/ctn-202109-figure2.png?itok=qgtdQSze)
RISs, in a Nutshell…
In this context, RIS has emerged as a new enabling candidate future wireless technology for shaping and controlling the electromagnetic waves between transmitters and receivers, in a dynamic and goal-oriented way, thus turning the wireless environment into a service [11]. As illustrated in Figure 1, an RIS is a planar surface that consists of an array of passive scattering elements, each of which can independently impose the required phase shift, and possibly an amplitude gain, on the incident electromagnetic waves. By carefully adjusting the phase shifts (and the amplitudes) of all the scattering elements, the reradiated electromagnetic waves can be shaped to propagate towards specified directions. Broadly speaking, an RIS is a planar (or, more in general, conformal) structure that is engineered to have properties that enable one to dynamically control the electromagnetic waves, via, e.g., signal reflections, refractions, focusing, collimation, modulation, and their combinations.
As shown in Figure 1, a typical RIS mainly consists of a planar surface and a controller. The planar surface is usually made of a single or, in general, a multiple layer structure. Often utilized three-layer structures are constituted by an outer layer with a large number of scattering elements printed on a dielectric substrate, which directly act on the incident electromagnetic waves; a middle layer that can be a copper panel to avoid signal/energy leakage; and a third layer that includes the necessary circuitry for controlling the scattering properties of the RIS elements. Figure 1 shows an example of the conceptual structure of each scattering element, in which a positive-intrinsic negative (PIN) diode enables the reconfigurability of the surface. By controlling the voltage through the biasing line, the PIN diode can switch between “on” and “off” modes, as shown in the equivalent circuit of the figure, which can realize a phase shift difference of 180 degrees. To increase the number of phase shift levels, several PIN diodes need to be integrated on the surface for each RIS element. Figure 2 reports an example of manufactured RIS that enables a continuous control of the phase of the reradiated electromagnetic waves by using four varactors for each available RIS element.
RISs, Pros, & Cons…
An RIS can be implemented using mostly passive elements, without requiring high-cost active components, such as power amplifiers, thus possibly resulting in a low implementation cost and energy consumption. An RIS is not expected to possess sophisticated signal processing capabilities, but only the necessary low-power electronic circuits for enabling it reconfigurability and hence controlling the propagation environments. This may in turn result in an easier and more flexible deployment, with the possibility of RIS-equipped nodes to take any shapes and to be even integrated onto objects (e.g., walls, buildings, lamp posts, and indoor and outdoor advertising street furniture). Thanks to these features, an RIS is often referred to as a “nearly passive” device and hence it is unlikely to increase the exposure to electromagnetic fields. These associated characteristics suggest that an RIS may be considered as a sustainable and environmentally friendly technology solution. The absence of power amplifiers and digital signal processing capabilities naturally pose some design challenges, such as the impossibility of on-board channel estimation, signal regeneration, and amplification, which are currently being tackled by wireless researchers and engineers [12], [13]. In general, these design features of an RIS result in an inherent trade-off between the coverage range of the surface, its size, and the number of RIS elements that need to be deployed on it: The larger the desired coverage range is, the larger the size of the RIS or the larger the number of RIS elements on the same surface area need to be. Surfaces with densely deployed RIS elements whose sizes and inter-distances are smaller than half of the wavelength pose implementation challenges as well, e.g., due to non-local mutual coupling effects among the RIS elements and the need of designing sub-wavelength scattering particles and tuning circuits. Table 1 offers a non-exhaustive and qualitative comparison between an RIS and other technologies that are widely employed in wireless communications and networks.
Technology/Concept | Characteristics | Key Differences to RIS |
---|---|---|
Reconfigurable Intelligent Surface | Intelligent radio surface whose response can be reconfigured using control signaling | -- |
Surface | Passive surface that follows Snell's law (for example, a metallic sheet) | Fully passive |
Intelligent (Engineered) Surface | Surface that can be appropriately engineered/designed to go beyond Snell's law | Not reconfigurable |
Repeater | Non-regenerative relay that can amplify and forward RF signals | It requires some active components, but with low control overhead |
Relay | Regenerative relays that can decode and forward RF signals | It requires significant active components and digital signal processing |
Massive MIMO | Antenna-integrated radio, typically comprising many transceiver chains | Fully active, full-stack cell |
From an implementation perspective, an RIS does not usually need active RF chains and only needs passive scattering elements and low-power electronics, which may potentially result in orders-of-magnitude lower hardware cost and energy consumption compared to 5G technologies based on active antenna arrays. The passive reradiation of an RIS mimics a full-duplex transmission mode that can be free of antenna noise amplification and self-interference. This may make an RIS more attractive than traditional active half-duplex relays that suffer from low spectral efficiency or full-duplex relays that require sophisticated techniques to cancel the self-interference out. Since an RIS is nearly-passive, it may be fabricated with light weight, low profile, and can be even made conformal to various objects. As a result, an RIS may be deployed in a wide range of scenarios, such as walls, ceilings, billboards, lampposts, and even on the surface of vehicles to support several applications for smart factories, stadiums, shopping centers, airports. Last but not the least, an RIS is often viewed as an energy-efficient auxiliary device that is transparent to the wireless users since it does not require modifications in the hardware configuration of the end-user devices. This offers high flexibility and compatibility with legacy wireless systems. As of today, however, the actual performance gains of RISs with respect to other widely deployed technologies, and the most promising use cases and applications for RISs are still under discussion. This is indeed one of the main objectives of currently ongoing collaborative research projects on this technology and of the recently established ETSI ISG on RISs. We will get back to this shortly…
RIS-empowered SREs
In wireless communication networks, RISs are intended to realize so-called programmable and reconfigurable wireless propagation environments, i.e., wireless environments that are not viewed and treated as random uncontrollable entities but become part of the network design parameters that are subject to optimization for supporting diverse performance metrics and quality of service needs, so as to fulfil the requirements of future 6G networks [8], [9], [12]. Recent applications of RISs in wireless communications include their use as nearly passive relay-type surfaces [14], signal-RF multi-stream multi-antenna transmitters [15], and reconfigurable ambient backscatters [16].
![Figure 3: Illustrative diagram of an RIS-aided smart radio environment. An RIS is viewed as a new type of system node with a smart radio surface technology whose response can be adapted to the status of the propagation environment through control signaling (courtesy of the ETSI-ISG on RISs [4]).](/sites/default/files/styles/400wide/public/images/2021-2021-09/ctn-202109-figure3.png?itok=Vj4e3wk9)
As illustrated in Figure 3, capillary deployed RISs may turn a wireless environment into a service by controlling the electromagnetic waves between specified transmitters and receivers, in a dynamic and goal-oriented way. This has motivated wireless researchers to evaluate new potential use cases targeting at (i) enhancements of various system key performance indicators, such as capacity, coverage, positioning, sustainability, and security and (ii) enabling new wireless technology applications, such as sensing, localization, wireless power transfer, and ambient backscattering. Broadly speaking, an RIS is viewed as a new system node that is dynamically configured by a system controller, turning a wireless environment from a passive to an intelligent actor such that the channel becomes programmable: An SRE. This trend is currently challenging basic wireless system design paradigms and is creating innovation opportunities which may progressively impact the evolution of wireless system architectures, access technologies, and networking protocols.
It is well understood that the RIS technology is not the panacea of future telecommunication standards. Several challenges need to be tackled for the possible deployment and integration of this technology in future wireless networks. At best, RISs can enhance current wireless networks that are designed based on active infrastructure elements and may turn future wireless systems into a hybrid network that comprises active and passive network elements and components, which are co-designed and co-work in an intelligent way for achieving a sustainable capacity growth at a low and affordable cost, implementation complexity, power consumption, in a sustainable environmentally friendly manner.
Collaborative Research Efforts and IEEE COMSOC Initiatives
RISs have attracted a lot of attention in the research community globally, including, noticeably, Europe, USA, and China. There exist several collaborative research and innovation projects on RISs and SREs. A non-exhaustive list of projects funded by national and supranational agencies is given in Table 2. By considering only the projects reported in the table, we can see that more than 20 million euro have been allocated by funding agencies to this technology, without considering industry-funded projects.
Title | Main Objectives and Outcomes | Start-End Months | Funding Agencies | Overall Budget |
---|---|---|---|---|
Manipulating Terahertz Waves Using Three-Dimensional Metamaterials | Manipulation of the polarization of THz waves using passive or active metamaterial based devices. | September 2012-August 2016 | NSF | $267,000 |
VisorSufg – A hardware Platform for Software-driven Functional Metasurfaces | Joint hardware and software designing for RIS with two experimental prototypes proposed. | January 2017-December 2020 | Horizon 2020 | €5,748,000 |
Assessing the Feasibility of Programming the Ambient Wireless Environment | Exploring ways of changing the perceived channel along the wireless link to create more favorable conditions for communication. | May 2018-April 2020 | NSF | $191,278 |
Liquid Metal Tuned Flexible Metasurfaces | Deployable, transportable and conformable meta-surfaces that can be tuned on-demand and in real-time for radio signals. | September 2019-August 2022 | NSF | $290,999 |
Artificial Intelligence Aided D-band Network for 5G Long Term Evolution (ARI-ADNE) | Merging a novel high-frequency advanced radio architecture with an artificial intelligence network processing and management approach into a new type of intelligent communications system beyond 5G. | November 2019-October 2022 | Horizon 2020 | €5,968,393.75 |
Scaling WLANs to TB/sec: THz Spectrum, Architectures, and Control | A first-of-its-kind pixelated metasurface waveguide to dynamically steer a THz beam via electrical switching of the meta-elements. | July 2020-June 2025 | HSF | $171,453 |
Enabling Seamless Coexistence between Passive and Active Networks using Reconfigurable Reflecting Surfaces | Developing a novel framework that enables seamless co-existence among multiple passive and active wireless systems, by exploiting the RIS concept to suppress interference at the wireless receivers. | October 2020-September 2023 | NSF | $320,000 |
Future Wireless Communications Empowered by Reconfigurable Intelligent metamaterials (META WIRELESS) | Enabling the manipulation of wireless propagation environments by reconfigurable intelligent surfaces. | December 2020-November 2024 | Horizon 2020 | €3,995,128.44 |
Reconfigurable Intelligent Sustainable Environments for 6G Wireless Networks (RISE-6G) | Several of studies, engineering and standardization practices which can bring the technically advanced vision on RIS into industrial exploitation. | January 2021-December 2023 | Horizon 2020 | €6,499,613.75 |
Harnessing multipath propagation in wireless networks: A meta-surface transformation of wireless networks into smart reconfigurable radio environments (Pathfinder) | Setting the theoretical and algorithmic bases of RIS-empowered wireless 2.0 networks that will lead to further transformations of wireless networks. | May 2021-April 2023 | Horizon 2020 | €184,707.84 |
Surface waves in smart radio frequency environments (Surfer) | Pioneer the theoretic foundation and experimental validation of surface wave communications for indoor communications. | February 2022-January 2024 | Horizon 2020 | €184,707.84 |
In the summer of 2020, the IEEE Communications Society launched several seed initiatives for promoting and supporting research activities in the emerging area of RIS-empowered SREs. This includes a Special Interest Group (SIG) within the Wireless Communications Technical Committee [18], an SIG within the Signal Processing and Computing for Communications Technical Committee [19], an Emerging Technology Initiative (ETI) on RIS [20], which was established by the IEEE Communications Society Emerging Technologies Committee (ETC), and a Best Readings in RISs [21]. These initiatives have fueled several opportunities for nurturing the RIS technology, which encompass the first special issue published in the IEEE Journal on Selected Areas in Communications in November 2020 [22], the latest forthcoming multidisciplinary special issue in the Proceedings of the IEEE [23], the first thematic workshop on RISs at the 2020 IEEE Global Communications Conference [24], a special workshop on RISs at the 2022 IEEE International Conference on Communications [25], and the first dedicated Selected Areas in Communications symposium at the 2022 IEEE Global Communications Conference [26].
Furthermore, the RIS technology is featured as a key technology trend in the International Telecommunication Union Radiocommunication Sector (ITU-R) - IMT-2030 Future Technology Trends report, and early attempts to bring it for exploratory studies in Standards Development Organizations including the 3rd Generation Partnership Project have recently been made.
The Road to Standardization: ETSI SIG on RISs
While all these extensive research efforts on RISs are ongoing, the global standardization of this technology is still at its very early stages [17]. There are many technical challenges that need to be adequately addressed before the RIS technology may be adopted and integrated into future telecommunication standards towards its eventual commercialization.
On June 30th, 2021, an initiative to constitute a new ETSI ISG on RISs was approved by the ETSI Director-General. This initiative, jointly led by InterDigital Europe (UK) and CNRS-UPSaclay (France) along with other founding members (British Telecommunications, CNIT, IMEC, NPL, NEC Europe, UK DCMS, University of Oulu, University of Surrey, and ZTE Corporation), was formed to coordinate and streamline pre-standardization research efforts on this emerging technology [4]. The mission statement of the ETSI ISG on RISs reads as
“To provide an opportunity for ETSI members to coordinate their pre-standards research efforts on RIS technology across various EU/UK collaborative projects, extended with relevant global initiatives, towards paving the way for future standardization of the technology”
The ETSI ISG on RISs, which will kick start on September 30th, 2021 and will run for a duration of two years, will focus on pre-standardization work, including the definition of use cases, application scenarios, and relevant system requirements. The activities of the ISG on RISs will be complementary to the existing work of ETSI. Relationships with other ETSI bodies and industrial organizations will be established to avoid duplications, to maximize synergies, and to ensure the broad industrial adoption of the RIS technology. The outcomes of the ETSI ISG on RISs will be made available through publicly accessible deliverables for wide dissemination and feedback [4].
RIS, Quo Vadis?
It is difficult to predict. Many research and deployment challenges need to be addressed, including the realization of sub-wavelength digitally controllable metamaterials operating at 6G frequencies, the design of efficient channel estimation algorithms, the definition of efficient control channels, and the conception of interference management schemes and communication protocols, just to mention a few. The ETSI SIG on RISs will kickstart on September 30th, 2021 and will run for two years. At the same time, the major European-funded projects on RISs will end by 2023 and 2024. Hopefully, we will be able to answer this question in the coming couple of years…
References
- C. L. Holloway et al., “Reflection and transmission properties of a metafilm: With an application to a controllable surface composed of resonant particles,” IEEE Trans. Electromagn. Compat., vol. 47, no. 4, pp. 853–865, Nov. 2005.
- E. F. Kuester et al., “Averaged transition conditions for electromagnetic fields at a metafilm,” IEEE Trans. Antennas Propag., vol. 51, no. 10, pp. 2641–2651, Oct. 2003.
- L. Subrt, D. Grace, and P. Pechac, “Controlling the short-range propagation environment using active frequency selective surfaces,” Radioengineering, vol. 19, no. 4, pp. 610–615, Dec. 2010.
- https://www.etsi.org/committee/1966-ris
- C. Liaskos, A. Tsioliaridou, A. Pitsillides, S. Ioannidis, and I. F. Akyildiz, “Using any surface to realize a new paradigm for wireless communications,” ACM Commun., vol. 61, no. 11, pp. 30–33, Nov. 2018.
- M. Di Renzo et al., “Smart radio environments empowered by reconfigurable AI meta surfaces: An idea whose time has come,” EURASIP J. Wireless Commun. Netw., vol. 2019, May 2019.
- Q. Wu and R. Zhang, “Towards smart and reconfigurable environment: Intelligent reflecting surface aided wireless network,”, IEEE Commun. Mag. vol. 58, no. 1, pp. 106- 112, Jan. 2020.
- M. Di Renzo et al., “Smart radio environments empowered by reconfigurable intelligent surfaces: How it works, state of research, and the road ahead,” IEEE J. Sel. Areas Commun., vol. 38, no. 11, Nov. 2020.
- C. Pan et al., “Reconfigurable intelligent surfaces for 6G systems: Principles, applications, and research directions,” IEEE Commun. Mag., vol. 59, no. 6, pp. 14-20, June 2021.
- R. Fara et al. “A prototype of reconfigurable intelligent surface with continuous control of the reflection phase,” arXiv:2105.11862, 2021.
- E. Calvanese Strinati et al. “Wireless environment as a service enabled by reconfigurable intelligent surfaces: The RISE-6G perspective,” EuCNC/6G Summit, pp. 562-567, June 2021.
- Y. C. Liang et al., “Reconfigurable intelligent surfaces for smart wireless environments: Channel estimation, system design and applications in 6G networks,” Science China Information Sciences, vol. 64, no. 10, pp. 1-21, 2021.
- K. Zhi et al. “Two-timescale design for reconfigurable intelligent surface-aided massive MIMO systems with imperfect CSI,” arXiv:2108.07622, 2021.
- M. Di Renzo et al., “Reconfigurable intelligent surfaces vs. relaying: Differences, similarities, and performance comparison,” IEEE Open J. Commun. Society, vol. 1, pp. 798-807, 2020.
- Q. Li, M. Wen, and M. Di Renzo, “Single-RF MIMO: From spatial modulation to metasurface-based modulation,” IEEE Wireless Commun., arXiv:2009.00789, 2021.
- R. Fara, “Reconfigurable intelligent surface-assisted ambient backscatter communications - Experimental assessment,” IEEE ICC Workshops, pp. 1-7, 2021.
- R. Liu et al. “A path to smart radio environments: An industrial viewpoint on reconfigurable intelligent surfaces,” arXiv:2104.14985, 2021.
- https://sites.google.com/view/ieee-comsoc-wtc-sig-rise
- https://spcc.committees.comsoc.org/special-interest-groups/sig-reflections/
- https://riseti.committees.comsoc.org/
- https://www.comsoc.org/publications/best-readings/reconfigurable-intelligent-surfaces
- https://www.comsoc.org/publications/journals/ieee-jsac/cfp/wireless-networks-empowered-reconfigurable-intelligent-surfaces
- https://proceedingsoftheieee.ieee.org/upcoming-issues/reconfigurable-intelligent-surfaces/
- https://globecom2020.ieee-globecom.org/workshop/ws-03-reconfigurable-intelligent-surfaces-wireless-communication-beyond-5g
- https://icc2022.ieee-icc.org/program/workshops#ws-1
- https://www.comsoc.org/conferences-events/ieee-global-communications-conference-2022
Statements and opinions given in a work published by the IEEE or the IEEE Communications Society are the expressions of the author(s). Responsibility for the content of published articles rests upon the authors(s), not IEEE nor the IEEE Communications Society.